The Full Story
About
This is your About Page. It's a great opportunity to give a full background on who you are, what you do and what your website has to offer. Double click on the text box to start editing your content and make sure to add all the relevant details you want to share with site visitors.
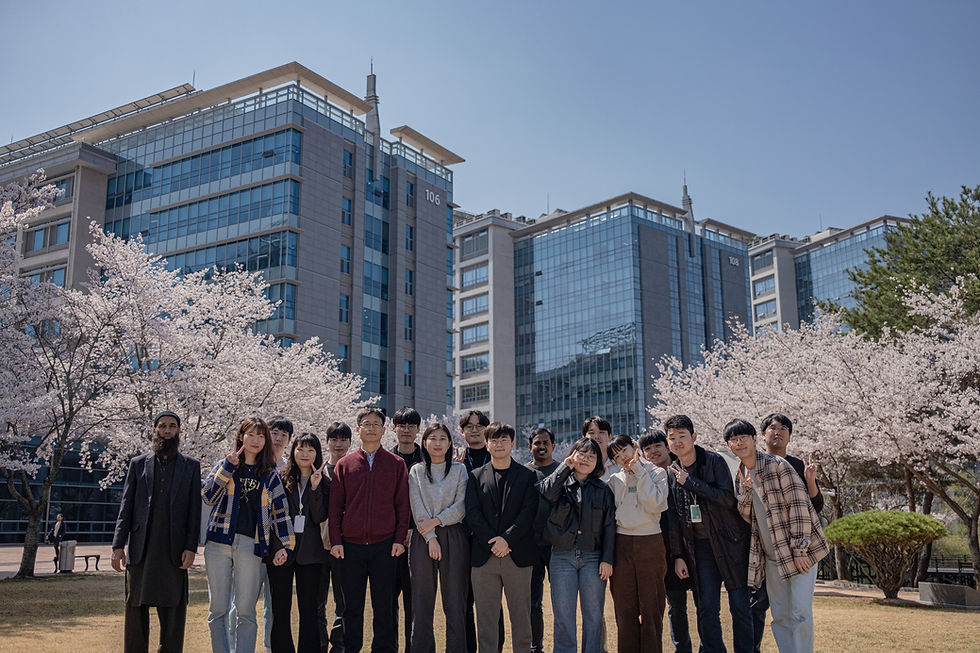
Our Laboratory
Electronic devices such as TV, smartphone, and monitor have become indispensable for modern life. These electronic devices utilize unit elements based on semiconductor materials, mostly inorganic materials like silicon. Inorganic materials have been essential for the development of electronic products due to their superior performance and reliability in aspect of device operation. However, future electronic devices need to offer greater diversity and undergo continuous development to satisfy new demands for wearable electronic devices. To achieve these, materials that possess not only performance and reliability but also flexibility, lightness, and cost-effectiveness are necessary. Organic-based semiconductor materials are attracting attention in this regard. In addition, they provide the advantage of easy control over physical and chemical properties by adjusting the chemical structure.
Our "Polymer/Organic Semiconductor Laboratory (POSL)" is a research lab that develops semiconductor materials based on conjugated organic compounds. We synthesize a variety of conjugated polymers and small molecules required for next-generation electronic devices such as organic solar cells (OSCs), organic transistors, organic thermoelectrics, organic light-emitting diodes (OLEDs), and quantum dot light-emitting diodes (QD-LEDs). Primarily, we synthesize materials that control optical properties and energy levels through the combination of electron-rich donor and electron-deficient acceptor chemical structures. Through evaluating the electrical and optical properties of these materials, we conduct research to reveal the correlation between their chemical structure and electrical/optical characteristics. Furthermore, we are trying to continuously develop high-performance organic semiconductors through systematic chemical structure changes to improve device performance higher than the current level. Additionally, we are developing additives that can crosslink and pattern the organic semiconductor active layer, insulating layer, and electrode materials, which are components of electronic devices, without decreasing the electrical characteristics.
Development of photoactive materials
for efficient and stable organic solar cells
Solar cells are devices that directly convert solar energy into electrical energy, and they have attracted much attention as a renewable energy source due to the environmental pollution and global warming associated with the use of fossil fuels. Among solar cells, organic solar cells (OSCs), using conjugated polymers or small molecules as photoactive materials, have been actively researched due to their advantages of lightweight, semitransparency, flexibility, and solution-processibility compared to inorganic solar cells. The performance of OSCs is evaluated by a power conversion efficiency (PCE), which is consisted of three photovoltaic parameters: an open-circuit voltage (VOC), a short circuit current density (JSC), and a fill factor (FF). VOC represents the maximum voltage when no current is supplied. It mainly depends on the difference between the highest occupied molecular orbital (HOMO) energy level of a p-type material and the lowest unoccupied molecular orbital (LUMO) energy level of a n-type material. JSC indicates the maximum current density when the applied voltage is zero and is related to the light absorption of the photoactive materials. FF represents the ratio of the maximum power output that can be obtained from the solar cells to the product of VOC and JSC. It is influenced by several factors, including the morphology of the photoactive layer and the dynamics of charge recombination. By appropriately designing the photoactive materials, these photovoltaic parameters can be improved.
Currently, the use of non-fullerene acceptors (NFAs) based on Y6 (=IC2F-BEH-IC2F), which was reported in 2019 and used as an n-type material, has shown commercial potential exceeding 19%. However, the low device stability remains a major limiting factor for commercialization. Therefore, our group is focus on improving the stability of OSCs by designing the photoactive materials.
We synthesized a new asymmetric NFA, IPC-BEH-IC2F, by replacing an IC2F moiety which used as an end group in the IC2F-BEH-IC2F with a new electron-accepting unit, IPC moiety. Compared to other symmetric NFAs, namely IC2F-BEH-IC2F and IPC-BEH-IPC, the asymmetric IPC-BEH-IC2F based OSCs showed a higher PCE of 12.70% when blended with PBDB-T as a p-type donor, due to the achievement of a balance between VOC and JSC. Moreover, the asymmetric IPC-BEH-IC2F based OSCs exhibited superior long-term device stability than the symmetric NFA based OSCs because the IPC moiety promotes the intermolecular interactions, resulting in enhanced crystallinity in the photoactive layer. Notably, we demonstrated that the molecular packing of the photoactive layer affects the degree of silver (Ag) penetration from the electrode into the photoactive layer, which is one of major factors of the long-term device stability. Going forward, we design new photoactive materials to not only improve device performance but also device stability, further contributing to the advancement of the OSC field.
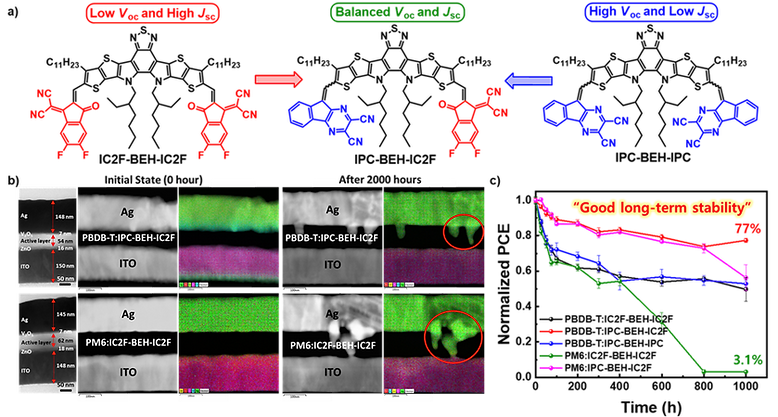
Figure1. a) Molecular structure of the materials utilized in this research b) monolayer image of the solar cell device fabricated using the developed materials in this research c) stability chart of the solar cells utilizing the newly developed materials in this research.
Development of high-performance
thermoelectric conjugated polymers
The field of thermoelectric materials has gained significant interest due to their unique ability to convert thermal energy into electrical energy. As the demand for wearable devices increases, research in thermoelectric materials that can generate electricity from body heat has garnered much attention. The performance of thermoelectric materials is expressed as the ZT value (ZT=α^2σTk^(-1)), where α, σ, T, and k are the Seebeck coefficient, electrical conductivity, absolute temperature, and thermal conductivity. Conjugated polymer-based thermoelectric materials have the potential to be used in the wearable devices because they are more flexible and have lower thermal conductivity than metal-based inorganic thermoelectric materials. However, the low electrical conductivity of conjugated polymers has made it difficult to commercialize them as thermoelectric materials.
Our research team have recently investigated the effect of molecular weight on the thermoelectric performance of the donor-acceptor type conjugated polymer, PDFD-T, based on the benzothiadiazole and dithienosilole moieties (Figure 2). Through controlling the molecular weight of PDFD-T, we have elucidated the influence of molecular weight on the thermoelectric performance of the conjugated polymers. Through various characterization techniques, we have discovered that higher molecular weight leads to better doping efficiency by dopants, which in turn facilitates the formation of polarons within the polymer chains. Moreover, we have observed that the decrease in the degree of crystallinity of the polymer after doping can be minimized by increasing the molecular weight. This phenomenon is found to have a profound effect on the charge carrier concentration and mobility of the doped conjugated polymer.
The electrical conductivity of PDFD-T was observed to vary according to the molecular weight, with the highest molecular weight polymer exhibiting a conductivity of 402.9 S·cm−1, while the lowest molecular weight polymer exhibited a conductivity of 39.5 S·cm−1. As a result, the PDFD-T polymer with the highest molecular weight exhibited the highest power factor of 101 μW·m-1·K-2, which is among the highest reported for conjugated polymer-based thermoelectric materials to date. Through a comprehensive analysis of the thermoelectric performance of the polymer with varying molecular weights, we emphasize the importance of molecular weight in optimizing the doping efficiency, electrical conductivity, and thermoelectric performance of conjugated polymers. Moreover, we highlight the potential of PDFD-T as thermoelectric materials. These findings pave the way for the development of high-performance thermoelectric materials based on conjugated polymers by elucidating the relationship between the chemical structure and molecular weight of the polymer and its thermoelectric performance. These efforts are expected to contribute to the advancement of reliable thermoelectric devices and technologies based on conjugated polymer materials.

Figure2. a) The chemical structure of conjugated polymers and the molecular weight. b) Electrical and thermoelectrical properties of PDFD-T depending on the molecular weight. c) Schematic image for the change in crystallinity of PDFD-T after doping. (L: low molecular weight, M: medium molecular weight, and H: high molecular weight)
Development of Transistors and Logic Devices
via All-Solution-Process
Solution processing is a technique employed in the manufacturing of electronic devices. It involves dispersing electronic component materials into a solvent and utilizing printing methods. This approach offers cost-effectiveness and scalability, presenting advantages over the use of expensive vacuum equipment. However, it also poses challenges, primarily related to the potential damage to the thin film layer of materials during the solution process, particularly when stacking different materials layer by layer.
The damage occurs due to the vulnerability of the initially formed material layer when subsequent layers are being formed. Additionally, the heat required to remove the solvent can cause further harm. Consequently, the manufacture of electronic devices through solution processing presents difficulties.
To tackle these challenges, we are actively developing various cross-linkers. Cross-linkers are substances that form covalent bonds between molecules within a material, acting as bridges that firmly hold the electronic device materials together. Our focus is on creating a novel multi-dimensional form of cross-linker called the multi-bridge cross-linker (nBx), which surpasses the existing 2Bx form with two cross-linking groups at each end of the molecule. By incorporating more cross-linking groups at the molecule's end, as seen in the 4Bx molecule, we can significantly enhance cross-linking efficiency. For example, the 4Bx molecule has demonstrated a doubling of cross-linking efficiency, increasing from 36% to 82%, compared to the existing 2Bx form.
Extensive research has been conducted on this 4Bx cross-linker, applying it to various materials such as n-type and p-type conjugated polymer semiconductors, PMMA for dielectric layers, and silver nanoparticles (Ag NPs) for electrodes. As a result, we have successfully produced high-resolution electronic devices that retain the unique electrical properties of the host materials, using only a small amount (1wt%) of 4Bx. Furthermore, we have confirmed the successful fabrication and normal operation of not only individual devices like transistors but also logic circuits through solution processing (Figure 3).
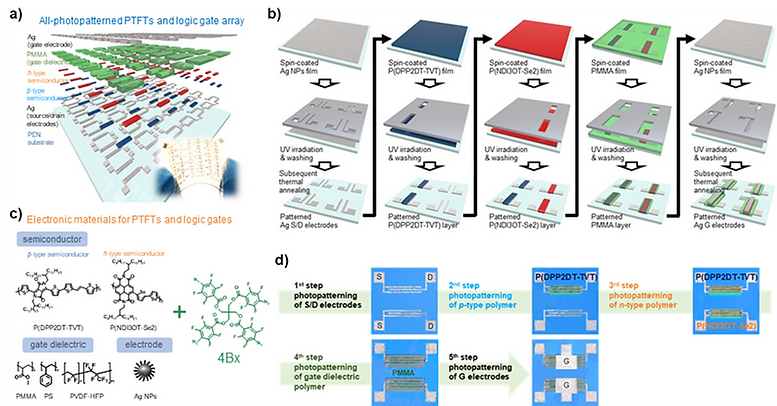
Figure 3. a) Schematic drawing and photographic image of all-photopatterned PTFTs and logic circuits fabricated on a plastic substrate by patterning of semiconducting channel, gate dielectric, and electrode materials. c) Chemical structures of electronic materials used in this study. d) Optical microscopy images of the component layers for a PTFT taken after each patterning step
Development of solution-processable patterning OLED and quantum dot light-emitting diodes
In addition to our previous work on cross-linkers and their applications in transistor and logic device development, our research also encompasses the production and investigation of light-emitting devices utilizing polymers and quantum dots.
Organic Light Emitting Diodes (OLEDs) have played a pivotal role in the display industry, serving as devices that emit light when electrons and holes recombine in the organic light-emitting layer, following injection from electrodes. Their intrinsic self-emissive nature eliminates the need for a separate backlight, offering the potential for thinner, lighter displays, reduced power consumption, and the ability to fabricate flexible devices. Achieving high-performance OLED-based displays with high resolution pixel-per-inch (ppi) requires the use of patterning processes. Consequently, developing manufacturing techniques that preserve the light emission efficiency of OLEDs during the patterning process and enhancing the overall stability of OLED materials for continuous operation are vital areas of research.
In our laboratory, we are conducting studies on the formation of patterned thin films and device characteristics using our multi-dimensional cross-linker (nBx) directly in the manufacturing process of organic light-emitting diodes (OLEDs) employing organic light-emitting materials. (Figure 2a, b) Through the utilization of our developed cross-linker (6Bx), we have successfully fabricated the emissive layer composed of light-emitting polymers and dopant/host materials widely used in OLEDs. By employing the solution process and appropriately adjusting the area ratio of consecutive light-emitting layers, we have improved an OLED device capable of displaying white light. (Figure 2c)
Quantum dots (QDs), emerging as next-generation materials in the display industry, are semiconductor materials with sizes on the nanometer scale, offering high luminous efficiency and color purity. Developing patterning technologies for high-resolution light-emitting layers that incorporate red (R), green (G), and blue (B) quantum dots within display pixels is a key research direction for the implementation of future quantum dot-based displays. (Figure 3b) However, the technology to pattern quantum dots according to their purpose without compromising luminous efficiency has yet to be realized.
To address this challenge, we have synthesized a new cross-linker capable of forming three-dimensional multi-bridge structures (up to 6Bx) by introducing isopropyl groups as an upgrade to existing cross-linkers. This innovation minimizes the risk of impairing the photonic and electrical properties of quantum dots, as it selectively cross-links only the "ligands" between quantum dots, without affecting the quantum dot core. (Figure 5a) Notably, our findings have shown improved device lifetime without decreasing light-emitting properties when compared to cross-linker-free light-emitting devices. Additionally, we have achieved the implementation of an RGB pattern using quantum dots with a remarkable line width of 1 μm. The patterning process technology utilizing this cross-linker holds significant potential for industrial impact, as it can be seamlessly integrated into the widely utilized photolithography equipment in the semiconductor and display industries. (Figure 5d)
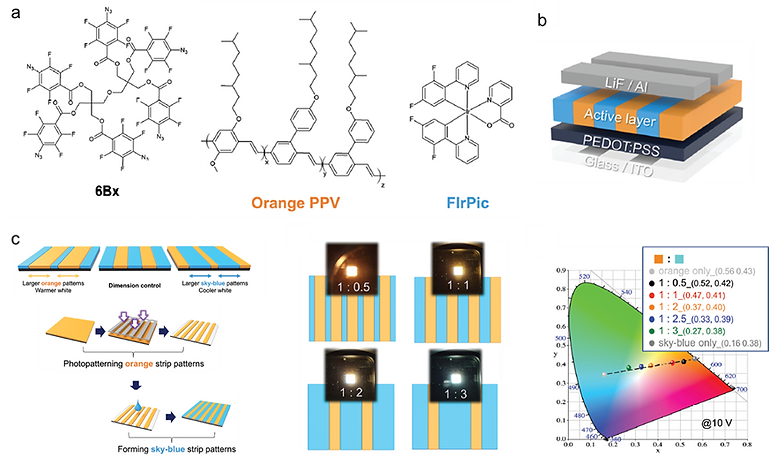
Figure 4. a) Chemical structure of 6Bx-crosslinker, orange PPV copolymer, and FIrPic. B) Schematic of a white OLED device based on the two-color strip-pattern. c) (From left) Schematic of the processing steps to form the two-color strips, Schematic of color-temperature control achieved by changing the areal ratio between the orange and sky-blue strip-patterns, Commission Internationale de l’éclairage (CIE) 1931 color coordinates of the emission from WOLEDs comprising two-color strip-patterns of varying areal ratios; measured at 10 V. Corresponding color coordinate values are inserted inside the legend.
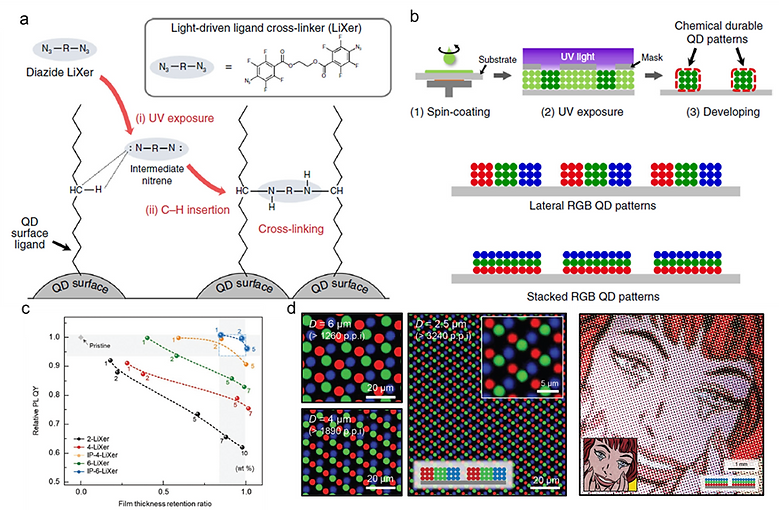
Figure 5. a) Schematic description of the ligand crosslinking process between neighboring QDs based on the C–H insertion reaction of the nitrene moiety of crosslinker. b) Schematic description of the photo-patterning processes of QDs using crosslinker. c) Relative PLQY plotted as a function of film thickness retention ratio, for QD films crosslinked with different branched crosslinker. d) Fluorescence microscopy images of RGB QD patterns fabricated by repeated photopatterning. Happy Tears” by Roy Lichtenstein (the original image is in the inset). © Estate of Roy Lichtenstein/SACK Korea 2022; reproduced with permission.